Introduction
The British Petroleum oil spill in the Gulf of Mexico, and the resulting damage to marine life and the coastal shoreline as the spill could not be quickly and effectively contained and remediated, serves as a reminder that our fragile aquatic ecosystems are not well protected from such accidents. The reason that an oil spill (an example is given below) spreads so rapidly on the sea surface is explained in Fig. 1. Oil spreads rapidly because the tension of the air/sea interface is much larger than the sum of the air/oil and oil/sea tensions at the slick periphery. This allows the seas surface to pull at the spill perimeter, drawing the spill out into a thin floating lens.
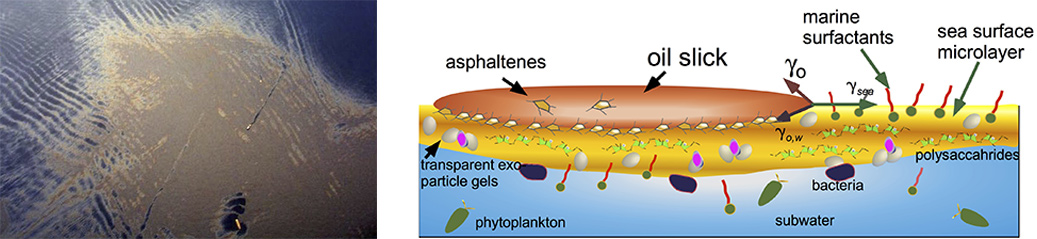
Figure 1: A maritime oil spill and the forces acting on the floating lens of the spill as it resides on the seas surface microlayer. The microlayer contains polysaccharides and a bacterial community unique to the layer, the neuston.
To date, the two principal spill response methods are: (i) the use of floating barrier booms to encircle and contract the spill, so that it may be removed by mechanical skimming or in-situ burning, and (ii) the aerial spraying of surfactants onto the spill to cause the oil slick to emulsify into small droplets that are carried away from the site or are broken down by microbes. Taken together they are an inadequate toolbox: Booms are difficult to deploy, and dispersants do not really remove the oil from the marine biota, and the effect of currently utilized dispersants on marine life is under dispute.
Our Research
The object of our research is to develop “chemical herding” as an effective oil spill response. In this method, surfactant is aerially sprayed around the spill, forming an encircling monolayer on the sea surface which lowers the large surface tension of the air/sea surface uncontaminated by the oil. The reduction in the surface tension causes the floating oil layer to retract and thicken to the point where it can be ignited and burned away (Fig. 2). The three challenges to designing a chemical herding formulation are : (I) the surfactant molecules are eco-friendly, (ii) the herders must significantly lower the sea surface tension and (iii) the herding mololayer which encircles the spill should be resilent and remain intact under interfacially turbulent conditions caused by wave action. The last requirement entauls designing herding systems to have a large dilatational viscosity to dampen the surface waves.
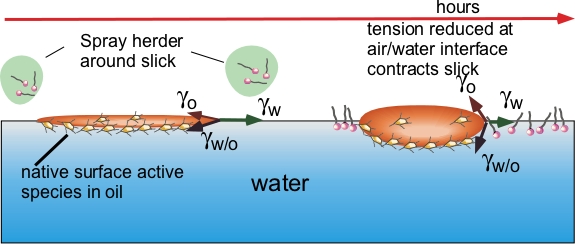
Figure 2: The chemical herding process for oil spill remediation
Our central idea is to design the herder as a mixture consisting of two eco-friendly surfactant components: The first is a glycolipid, which, because of its large sugar polar group forms entanglements in the sublayer of water underneath the interface. The entanglements can create a large dilatational viscosity which can dampen surface waves and increase the effectiveness of chemical herding, particularly if the sugar residues can bond with the polysaccharides which are present in the sea surface microlayer (see Fi. 1 and 4). We use in particular the glycolipid MGDG a major component of the thylakoid membranes in the chloroplasts, the organelles in all green plants (abundant in the marine biota) which are responsible for photosynthesis (Fig. 3). By themselves, glycolipids cannot pack into a dense, surface tension lowering monolayer because of the large sugar polar group. The second component in the formulation is responsible for the tension reduction by synergistically inserting into the glycolipid monolayer to form a dense layer. For this second component, we use phytanic acid (Fig. 3), an isoprenoid with a branched hydrocarbon tail. Phytanic acid is chosen because the unique branched structure of its phytanyl hydrocarbon tail readily allows the molecule tp insert itself into the MGDG-rich thylakoid bilayer membrane. In the thylakoid membrane the phantanyl tail is conjugated to the chlorophyll molecule, and anchors chlorophyll to the membrane so the chlorophyll can harvest sunlight and transfer the energy to the photosynthetic reaction center proteins in the membrane.
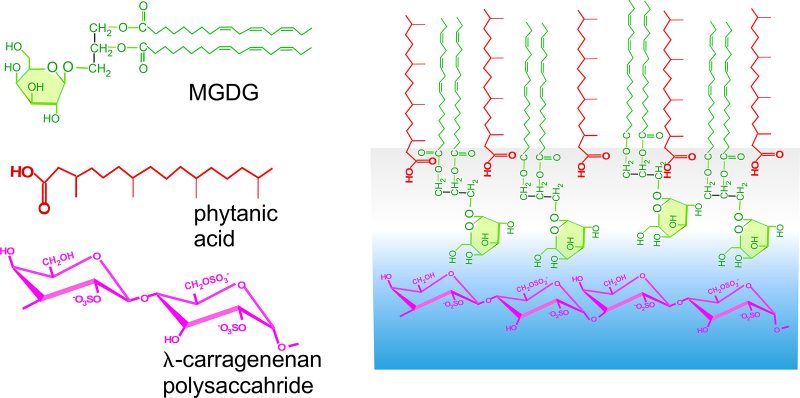
Figure 3: The components of the chemical herding formulation and the mixed monolayer at the sea surface.
Lab scale experiments (below) show the effectiveness of the MGDG monolayer alone at reducing the sea surface tension in experiments on compressing a floating oil lens on a small rectangular trough (Fig. 4). In these experiments, the lens is initially enclosed by a teflon ring and the surfactant is added to the surrounding surface. After the ring is lifted, the MGDG compresses the layer as recorded by a camera above the lens. The reduction in area is computed, and plotted in the figure below for two conditions: First in the absence of a marine polysaccharide, and then in the presence of the biopolymer (here lambda carrageenan, Fig. 4, is used). The greater effectiveness in the presence of the lambda carrageenan indicates the MGDG recruits the biopolymer to the interface, because in the absence of MGDG the carrageenan does not significantly reduce the sea surface interfacial tension.
Figure 4: Lab-scale experiments on chemical herding. The movies show the immediate spreading of oil on the surface in the absence of the herding, and in the presence of MGDG.